Sending bread boards to the heart failure wards
This submission by Julian FR Paton FRSNZ captures a 12-year research effort to create a new cardiac pacemaker based on the way nature has been pacing the heart for 431 million years.
Smart watches often include a readout of ‘HRV.’ HRV stands for heart rate variability, which is an index of good health and physical fitness. Athletes will have a relatively high HRV, which can be further elevated on training. In contrast, low HRV is prognostic for cardiovascular disease, is absent in patients with heart failure and is associated with sudden cardiac death. So, what causes HRV?
In 1733 Reverend Stephen Hales first found that heart rate rises on inhalation and slows during exhalation meaning that your breathing is coupled via the autonomic nervous system to modulate the rate of your heartbeat. This breath-by breath variation in heart rate, which is termed respiratory sinus arrhythmia (or RSA), is the most prominent frequency within HRV. Remarkably, RSA has been preserved since the Cambrian period when it first appeared in primitive cartilaginous fish where buccal pumping was coupled to heart rate. So, given its evolutionary longevity it must be good for something, but what?
To address this, we initiated a mathematical model and found that RSA preserved energy for the heart, which amounted to 35 calories per hour. Over a year this would accumulate to 300kcal, equivalent to the energy burnt by a human over 170 days. Given this, the hypothesis was generated that if RSA saves the heart energy, then a heart in failure, where the heart is depleted of energy, should thrive in the presence of RSA.
Reinstating RSA was not a trivial task, requiring the need to both sense the start and end of inspiratory and expiratory phases and to instantly pace the heart up and down accordingly by a pre-determined amount in real time as well as deal with changes in respiratory frequency and pattern that occur, for example, during swallowing, talking, yawning and sneezing. Digital circuitry was deemed too slow due to the time needed for digitizing the analog signals of respiration. So an analog device was built on a large bread board that was based on Hodgkin-Huxley-like neurones (Figure 1).
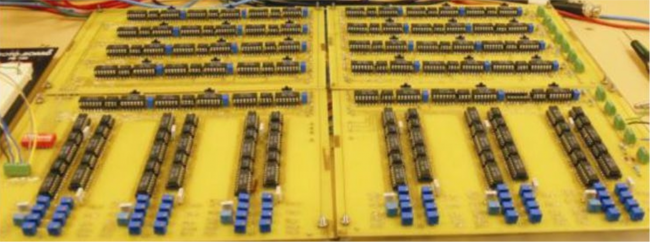
Figure 1: the breadboard analog pacemaker
Respiration was detected from the electrical activity of the diaphragm, which denotes the period of inspiratory time and used as a timing signal to the pacemaker. Optimisation was performed in rats and subsequently the electronic circuit was patented. The pacemaker was then tested on sheep in heart failure. For this we recorded cardiac output (volume of blood pumped per minute) as an index of heart pumping performance.
Initially, commencing RSA pacing in conscious instrumented sheep was hugely disappointing because cardiac output did not change. This was perhaps not surprising given that the energy saving predicted by our model indicated it would become more significant over time. Thus, we decided to continue pacing for longer in the hope that a miracle might happen.
Within three days cardiac output started to increase and by seven days it had plateaued off with an impressive increase of 1.2 litres/min reflecting a 22% improvement. This is at least three-fold the increase seen with current pacemakers in fully-medicated patients. Moreover, the sleep apnoeas that are associated with heart failure also reduced. Most remarkable was that RSA pacing had reverse re-modelled the heart such that its ultra-structure was similar to that of a healthy heart: first, the t-tubules, which are intracellular invaginations essential for getting calcium ions deep into the inside of the cells and become fragmented in heart failure, were repaired; second, the ryanodine receptors had re-associated with the repaired t-tubules (Figure 2). This is critical because the ryanodine receptor is the main regulator of intracellular calcium essential for contractility.
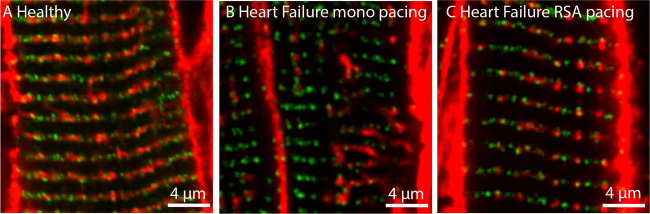
Figure 2: Reverse re-modelling heart failure by RSA pacing (red are the t-tubules and green are the ryanodine receptors).
Then followed commercialisation including creation of a spin out company (https://www.ceryxmedical.com/) and multiple rounds of funding raises to support a first-in-human trial. Given that the pacemaker remains on a breadboard and could not be implanted at this stage, it was decided that the trial should identify patients fitted with exteriorised pacemaker leads. Following coronary artery bypass graft surgery patients are fitted with such leads that remain in place for up to seven days post-operatively. Thus, the trial will pace these patients with RSA over one week post-operatively and this will take place at multiple sites within Aotearoa New Zealand commencing in the first quarter of 2023. If the spectacular response observed in sheep can be replicated in humans, then this discovery might revolutionise pacemakers globally.
It is clear that the heart never beats metronomically, yet this pattern is used by current pacemakers including those that respond to exercise. The natural breath-by-breath frequency modulation of RSA most likely triggers transcription that optimises cardiac contraction efficiency. What the transcription factors are and how frequency modulation activates them remains the next exciting scientific challenge that will further unravel the enigma of RSA.